"Peto's paradox: how big animals don't get cancer"
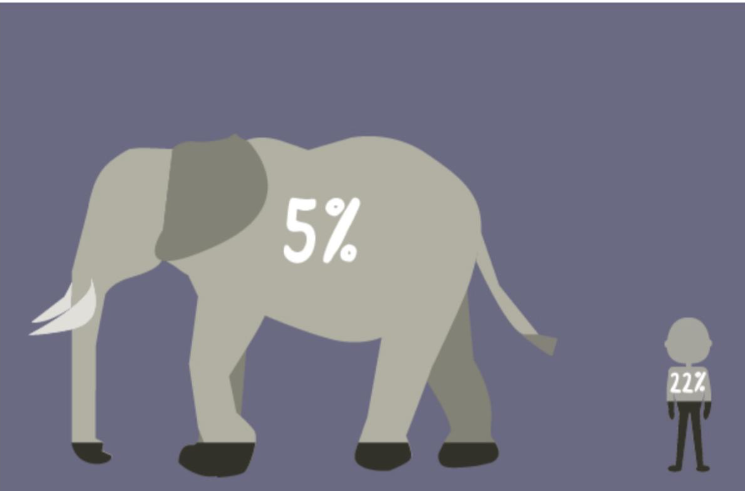
There are tens of trillions of cells in our body, occasionally some of them mutate and start to divide uncontrollably, stealing nutrition from normal functional cells. These are cancer cells, and according to the NHS, 50% of us will develop cancer at some point in our lives. Statistics show that taller people and men also have a higher possibility of developing cancer, the reason being these groups tend to have more cells which results in a larger number of net mutations, hence a larger number of cells transforming into malignant tumours. Following that logic, the number of cells in a mouse is 0.1% of a human being and the number of cells in a human being is 0.1% of a whale. Intuition tells us whales get much more cancer than humans and mice do not get cancer at all. Absurdly, the truth is the opposite, the fact that larger, long-lived animals rarely get cancer is known as Peto’s paradox.
Named after Richard Peto, the British epidemiologist posed this question in 1977 which still left scientists baffled. To this day, there’s still no unanimous agreement on this puzzle. Nevertheless, reasonable suggestions were made aspiring to solve the paradox.
One suggestion is the multiple copies of TSG (tumour suppressor genes) in large animals, these genes when activated, make cancer cells undergo apoptosis (programmed cell death) which can prevent the mutant cells from growing into anything harmful. The gene TP53 (which is a TSG) plays a key role in controlling cell divisions and helps prevent cancer development. Humans have one pair in each chromosome while African Elephants have 20 pairs. This means mutant cells need to escape 20 layers of protection to fully develop into a tumour in an African elephant. On top of that, mice with altered DNA of extra TP53 and Cdkn2A were shown with higher resistance to cancer. One problem with this solution is that other giant mammals like hippos and blue whales also only have one pair of TP53 yet they still have low cancer rates. Meaning there are more underlying factors which contribute to low cancer rates.
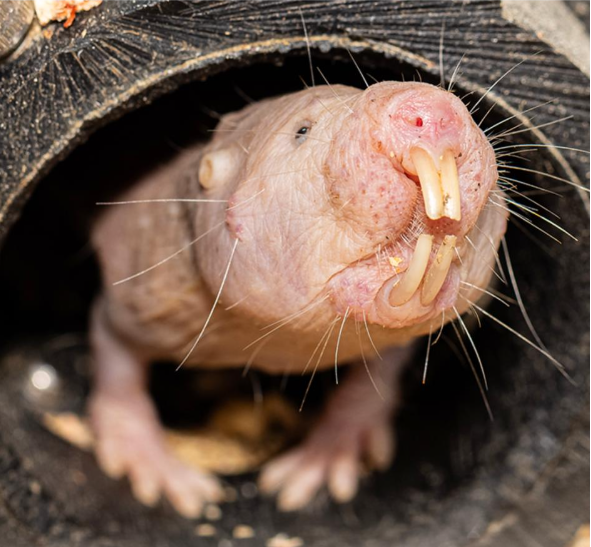
Another solution is contact inhibition, this mechanism prevents cells from dividing at high densities or coming into contact with each other. This stops the mutant cells from coming together and forming a big tumour. This was found in naked mole rats, despite being similar in size to mice, their lifespan is 28 years, seven times longer than the typical life expectancy of mice. The Cdkn2A gene mentioned earlier encodes a protein called p16, which plays a key role in developing hypersensitivity to contact inhibition in naked mole-rat cells. It has
been confirmed that naked mole-rat cells stopped dividing at much lower densities in culture than both human and mice cells, but it still hasn’t been testified at the organism level.
The other hypothesis is the formation of hyper tumours ( tumours that grow on tumours). These hypertumours come from the mutation of original tumours. As the neoplasm grows and builds new blood vessels around it (angiogenesis), some cells “betray” the host tumour and start to have a mind of their own. Meaning they would compete for resources with the original neoplasm, making use of the vascular infrastructure built by the original cancer cells. These “cheaters” can kill part or all of the host tumour, which controls the size and fitness of that region of the tumour. In theory, hyper tumours can develop in tumours of all animals, but there might not be time for hyper tumours to arise in smaller ones, as tumours of the same size can be much more lethal in a pika (150g) than a humpback whale (30 tons). Scientists proposed that there might be many tumours in larger animals but none of them dangerous enough to kill the organism, whereas a neoplasia the same size would be fatal in smaller animals.
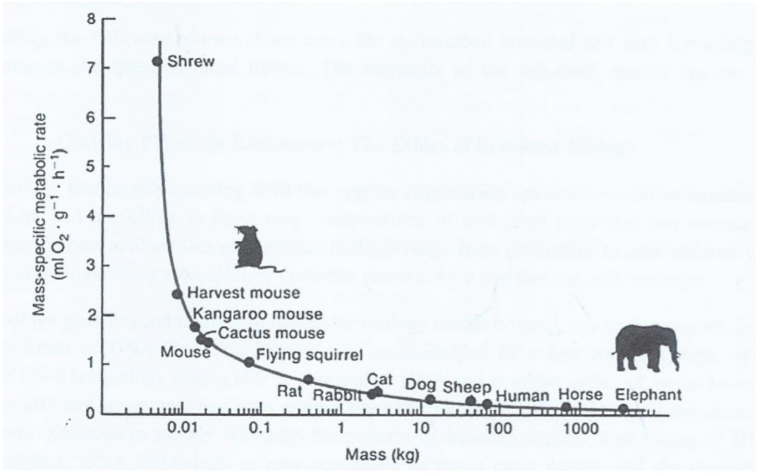
The last proposal I will talk about is the basal metabolic rate (BMR) of different organisms. The byproducts of metabolism contain substances that can damage
the DNA, these substances are called reactive oxygen species (ROS). The higher the BMR, the more damage is inflicted on DNA, including the destruction of oxidative repair genes. The basal metabolic rate per kilogram for mice is roughly 13 times the BMR/kg of elephants. As a consequence, less ROS is produced in bigger animals therefore less damage is done to their DNA, hence a lower mutation rate. Referring to the naked mole rats (although an anomaly in terms of size) mentioned earlier, their BMR is much lower than expected for a mammal so tiny, that this is thought to contribute to their resistance to malignant tumours.
There are also many other solutions to this paradox, like having shorter telomeres, a more efficient immune system and different tissue architecture. Evolution enabled large, long-lived animals to find ways of not getting cancer, otherwise they would just be walking blobs of tumours. The significance of further studies in this area limits not only to a deeper understanding of cancer prevention in large animals, but we can extend our discoveries to curing cancers in humans. However fully eradicating cancer as a species is not simple, higher cancer cure rate could come at a cost. For instance, better cancer treatment could affect longevity in other aspects, or slow down injury recovery. About 17% of humans die of cancer nowadays, and as one of the biggest causes of death, it is a global issue. By looking at how nature suppressed cancer for the past billions of years, we know we still have a long way to go.