The Forms of Silica: Why Are Crystals Like That?
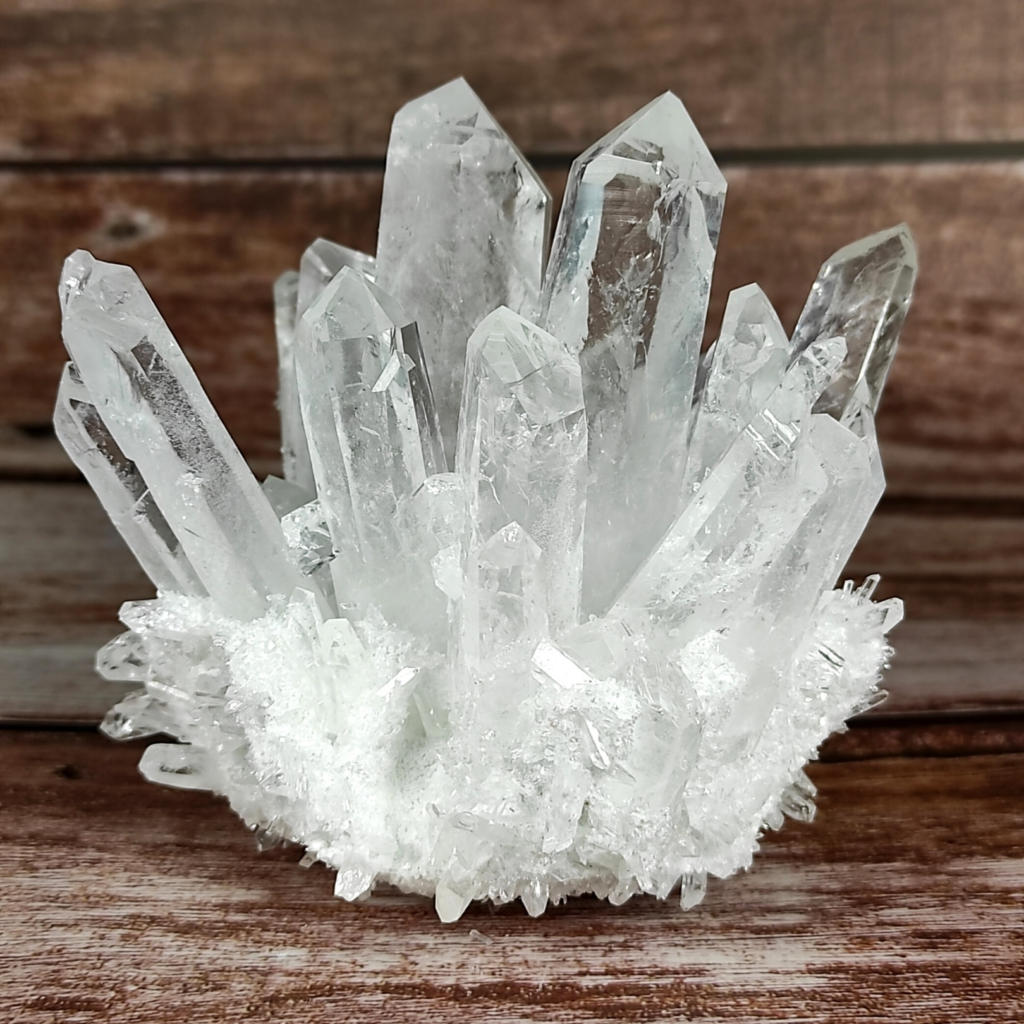
Amethysts, opals, quartz and sand. In our lives, we see all these things from time to time and we’re all pretty familiar with how they look. Amethysts are a deep purple where quartz are clear or white, precious opals have their ‘play-of-colour’ effect and sand is, quite plainly, just sand. These characteristics differentiate them from one another and tell us at a glance that each is unique, so what if I was to say that we’d gotten it wrong?
Every substance in the world can be broken down into elemental components, crystals are no exception, and it just so happens that if we look at each of these crystals on the molecular scale, they’re basically the same thing. Purple amethyst, clear quartz, iridescent opal and sandy sand are all just different forms of one thing, silicon dioxide (or SiO2).
Why silicon dioxide?
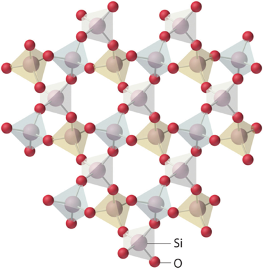
Opal and quartzes are by far not the only kinds of crystal with a shared formula – both sapphire and ruby are the chemical ‘corundum’ (Al2O3 ) – but with so many kinds of quartz in the world, ‘why’ is still an important question to ask. This comes down too two things you may remember from your GCSEs. Silicon is one of the most abundant chemicals in the world, making up almost 28% of the earth’s crust by mass, and is generally unreactive. Still, in the squash and squeeze of deep underground a chemical is forced to take up whatever form needs the least space. In crystals, this trait is referred to as something’s ‘packing efficiency’. Trapped by these hot, high-pressure conditions, silicon reacts with the doubly abundant oxygen (making up 46% of the earth’s crust by mass) to form dense, crystalline silicon dioxide. The reason there are 2 oxygens for each silicon is to do with their valance electrons, but I’m not going to bother with that here, you get it. Silicon is greedy that way.
After reacting with oxygen, silicon dioxide takes the form of a giant covalent lattice which, yes, say it with me chemists, means it’s held together by a lot of strong covalent bonds. These strong bonds are what make SiO2 crystals strong andrelatively inert, letting them stay in their crystalline forms until they’ve been pushed far enough up to the surface for us to find them, sell them at ridiculous prices and claim they have magic powers.
Why are quartzes clear?
Usually, if you had to imagine a crystal, you’d think of something sort of see-through and shiny, but crystalline formsare generally opaque. Whether a specific crystal is see-through or not is to do with it’s atomic structure and electrons. In a crystalline metal, there are many delocalised electrons available to absorb incident light, enter an excited energy state, and re-emit part of it as a reflection and part of it as heat. As all light is absorbed and none can pass through even the thinnest sliver of metal, metallic materials are perceived asopaque (that might sound a confusing right now, but i’ll go through it in a bit more detail later). In SiO2 however, distracted as they are by all the covalent bonding, the energy packed by visible light isn’t quite enough to get an electron going. Instead, light rays just pass straight through, leaving quartzes in the clear.
Another important thing to note here is that chemical formulasare quite often a gross oversimplification. If we weren’t to take them with a pinch of salt, then sand and quartz would have to be the exact same thing. Why, then, isn’t sand see-through too? That’s just because I lied a little earlier. Sand isn’t SiO2. It’s mostly SiO2, but out of the 2 x1019 atoms in each grain of sand, there’s a good fat chunk of other stuff going on that messes up all this see-through business. The impurities in sand are excited by light energy, absorb it, and re-emit it scattered out in all directions, leaving sand itself translucent (a sort of clear/opaque half-and-half). Simplifications like this crop up quite a lot in crystal science, but it’s all quite understandable. Impurities just make up so little of a materialand vary so much in concentration that trying to include them in a balanced empirical formula would drive all the scientists insane insane. Beach sand can be close to 100% SiO2, so all in all it’s a pretty fair title.
Quartzes can have impurities too (a lot of which are actual very important and will be talked about later), which can make them slightly cloudier, but purity isn’t the only thingmaking sand look opaque. A good, clear slab of crystal quartzhas a massive lattice and relatively even faces, meaning not too much light ends up scattered by messy edges. Sand on the other hand, has a much greater, much less polished, surface area for its volume and tends to come as tens of thousands of grains that are all scattering light off each other, resulting in their looking opaque as a group.
So what about amethysts then?
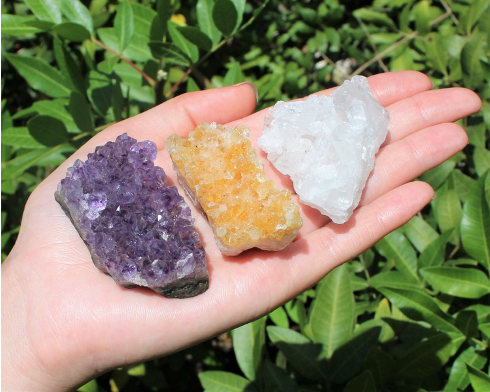
Onto the business of colours. Do you remember what I said about impurities? The colour of a quartz crystal is decided by a tiny tiny amount of transition metal impurities laced in it’s lattice. Amethysts, for example, contain trace amounts of iron.
Still, it’s not quite so simple. Both amethyst and citrine can be formed when iron ions replace a few silicons in the SiO2lattice, so how come one is purple and the other yellow? Well, it’s not exactly the element that matters. GCSE ion tests have taught us that all the iron ions form precipitates of different colours and that the Cu(I) and Cu(II) salts of copper even come out with slightly different colours in a flame test. This isbecause of electrons in a similar way to why quartzes are clear and metals are opaque.
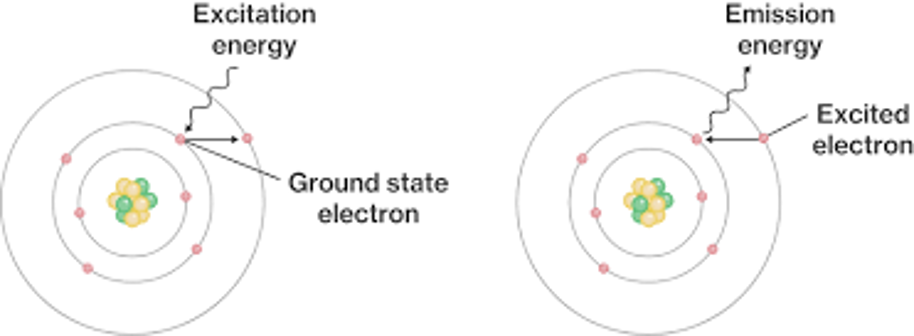
Imagine an electron. This electron exists in what is called its’s‘ground state’, which is wherever it likes to sit around the nucleus. When certain kinds of energy are fed into thiselectron, either as heat in a flame test or visible light, they areabsorbed, boosting it up into what is called an ‘excited state’ a little further out. In this state, the electron requires more energy to stay put, meaning it’s less stable and, eventually,it’ll give up and drop back down to where it came from, re-emitting the stored energy as heat and effectively removing it from the wavelengths of light reflected off or transmitted through a substance. We see the object, after all of this, as amix of all the colours that haven’t been absorbed, i.e: if the wavelengths for green, yellow, and orange have been absorbed, something ends up looking purple. Different ionshave different amounts and patterns of electrons so, ratherthan colour being decided by what element the impurities are, it is decided specifically by what ion they are.
Both citrine and amethyst start off with inclusions of the‘ferric’ Fe3+ ions, responsible for a yellow colour, but exposure to natural radiation can oxidise these further, forming Fe4+. These ‘ferryl’ ions are what let us see amethysts as purple.
D-block metals and colour
I mentioned above that it’s usually transition metal impurities that cause colour. There is a pretty good reason for this, and I’d recommend that you take a pause to look up ‘the d-sub shell’ if you don’t already know what it is.
The transition metals live in the D-block of the periodic table, but technically not all D-block elements are transition metals. To properly count, a metal has to form ions with a half-full d-orbital. By this logic, zinc and scandium, despite being D-block elements, are not transition metals.
An unpaired electron takes a lot less energy to excite than a paired one, and it just so happens that, though the energy of visible light is not enough to excite a lone d electron, certain wavelengths can excite a lone one. As we have already discussed, this is what results in colour. Most elements form ions by emptying out or filling a shell completely or else pair them up through covalent bonds, so it is unusual for non transition metals to have display bright colours.
Finally, opals
I know I’ve said a lot already, but bear with me, this is the last bit. So, amethyst, citrine, quartz do at least look sort of similar, so it’s not too great a shock that they’re chemically the same thing. Opals howeverare quite unique in their appearance, so are they really the same thing too?
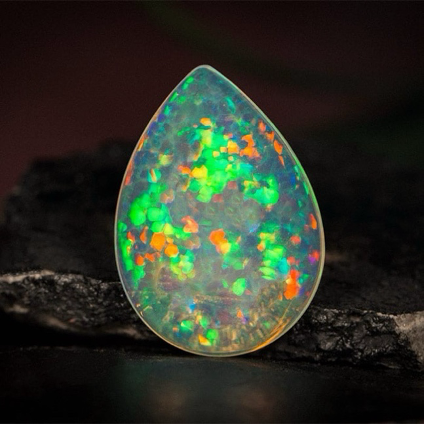
The simple answer is not quite. Opal and quartz have two crucial differences. One, opal is not technically a crystal. It’s a mineraloid, an amorphous form of hydrated SiO2 that lacks a regular crystalline structure. Second, opal actually has a slightly different chemical formula: SiO2.xH2O. This tells us that alongside the silicon dioxide opals contain some variable amount of H2O, usually between 3-21% of the stone’s mass.
On a very small scale, opals are actually little balls of this water mixed up with many more little balls of SiO2. If all the balls sit in a uniform pattern, a lattice is formed that channels light through the water balls as though they were very many slits in a diffraction grating, splitting apart all the wavelengths of ‘white’ lightwhich then interfere destructive or constructively, creating the glimmering hues we see. Stones with this arrangement are called ‘precious opals’ and are highly prized, whereas stones without this feature, and therefore without any play of colour, are called ‘common opals’.
The end
I’m not quite sure if I should call this physics or chemistry,and maybe that just goes to show how closely intertwined the two are. Either way, I definitely learned a lot while writingthis and, if you’ve made it this far with me, well done and thank you for being here!